Atomic force microscopy (AFM)-based single-molecule force spectroscopy enables a wide array of studies, from measuring the strength of a ligand-receptor bond to elucidating the complex unfolding pathway of individual membrane proteins. Such studies and, more generally, the diverse applications of AFM across biophysics and nanotechnology are improved by enhancing the force stability, force precision, and time resolution of bioAFM. For an advanced, small-format commercial AFM, we uncovered how these three metrics were limited by the cantilever itself rather than the larger microscope structure, and then describe three increasingly sophisticated cantilever modifications using a focused ion beam that yielded enhanced data quality. High-precision single-molecule studies of membrane proteins—the target for over 50% of current and future drugs—have lagged analogous studies of globular proteins due to such instrumental limitations. We revisited the unfolding of the canonical membrane protein bacteriorhodopsin (bR) embedded in its native lipid bilayer with a 100-fold improvement in time resolution and a 10-fold improvement in force precision. The resulting data revealed the unfolding pathway in unprecedented detail. Toward the broader goal of measuring quantitative energetics, we leveraged the rapid and reversible initial unfolding of bR to determine the free-energy change (ΔΔG0) for select point mutants. Hence, we established a platform for precisely quantifying membrane-protein energetics under native-like conditions. We then extended this assay to quantify a light-induced energetic change in bR’s photocycle by briefly illuminating the sample with sub-ms light pulses. I will conclude by discussing extensions of these enhancements to other diverse biomolecules, including nucleic-acid structures and globular proteins involved in pathogenesis.
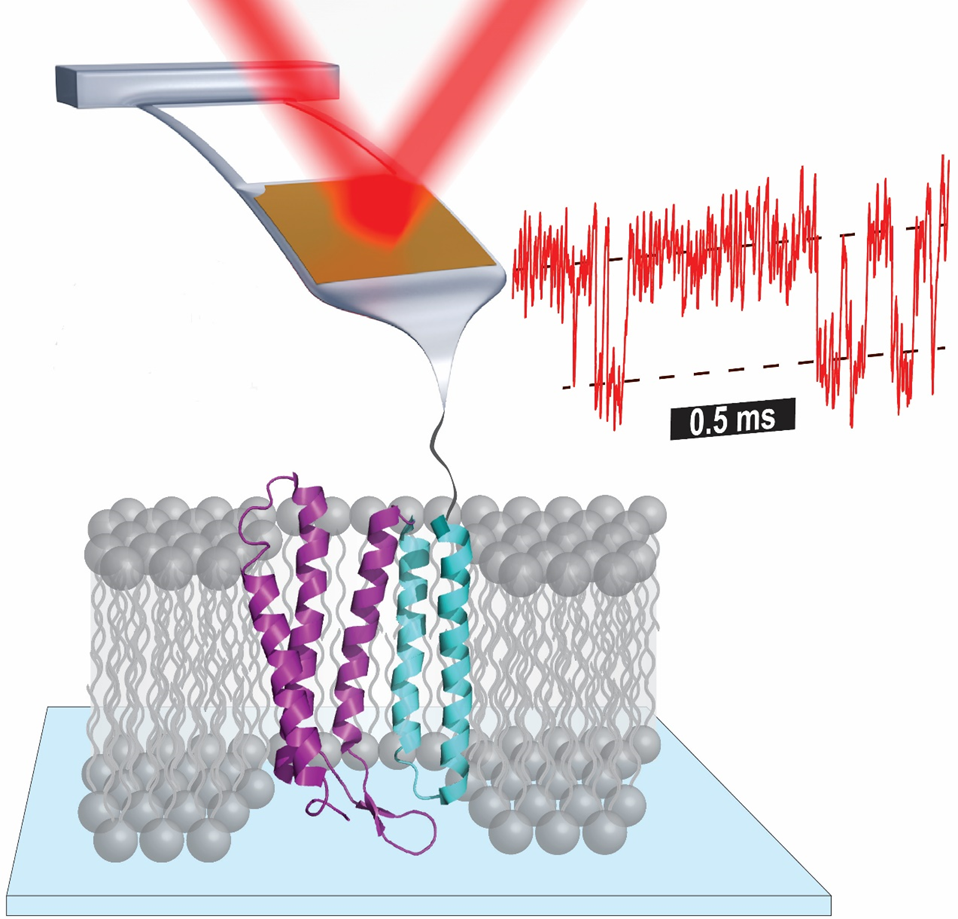